Bitesize and Sounds revision podcasts | Overview
Revise GCSE Biology by listening to these podcasts from Bitesize and BBC Sounds.
BBC Sounds is where you can catch the latest music tracks, discover binge-worthy podcasts or listen to live radio, all in one place.Listen on the BBC Sounds app when you're out and about, or listen at home as part of your revision.
Let Dr Alex Lathbridge guide you through the key facts about cell structure
Supercharge your revision with more podcasts for GCSE English literature and GCSE Biology
Episodes are roughly ten minutes long and there are up to nine episodes in each series.
Making notes as you listen can help you remember the key points before the exam.
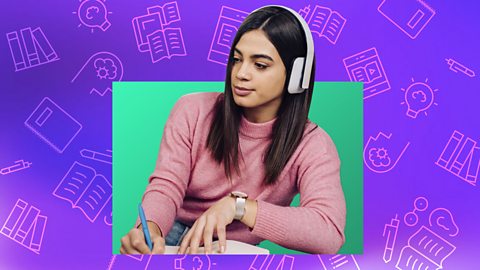
Episode 1 - Communicable diseases
Dr Alex Lathbridge breaks down the key facts about communicable diseases, looking at how they are spread and the four main types: viruses, bacteria, fungi and protists.
Hello, I’m Dr Alex Lathbridge and this is Bitesize Biology.
This series is about infection and response. We're going to take a deep dive into infection and how the body responds to it.
To start things off, we’re going to talk about communicable diseases, things like viruses, bacteria, fungi and, this might be new for you, protists.
But what about non-communicable diseases? Well we already did those in our series on the organisation of plants and animals. Maybe go back there and listen again if you’re not quite sure what they are.
Communicable diseases are spread between humans, or between humans and animals. They’re infectious, things like the flu virus and bacteria that cause food poisoning. Literally diseases that can be communicated from one to another.
The first thing you need to know is what a pathogen is, so grab a pen and write this down:
A pathogen is a microorganism that causes infectious, communicable diseases to plants and animals.
Pathogens have really simple life cycles. They infect a host (that’s the organism that pathogens live in or on), replicate or reproduce, and then move on to infect something else.
And the word pathogenic means that something can cause disease. There are five ways pathogens can spread (or transmit) from one organism to another:
Droplets. Pathogens travel in the air as droplets that can be breathed in. Like when you sneeze, virus droplets can be sprayed into the air, that others can breathe in and be infected with.
Water. Pathogens exist in water, so drinking infected water or washing in it can transmit the pathogen. For example, cholera is spread by drinking water.
Direct contact. Pathogens can be spread when contact is made by two individuals. Basically touching another person. So things like shaking hands, or sexual contact.
Food. Improperly cooked or reheated food can contain pathogens.
Vectors. These are organisms that spread disease, but they don’t get the disease themselves, like mosquitoes that spread malaria.
There are four different types of pathogens that we’re going to discuss today: viruses, bacteria, fungi and protists.
We’re going to start with viruses.
A virus is a short length of DNA or RNA, enclosed inside a protein shell.
These things are so simple that scientists right now debate whether they fit in the category of "this is a living thing" or "no, this is some kind of un-alive microscopic machine."
For your exams, you’d say viruses are not alive. And remember, they are not cells.
This means that there are different strains of viruses but not different species, because they’re not alive.
So how do they work?
Viruses can only survive by infecting a living host, which could be any organism on earth. This means no life form is safe from infection by a virus.
Inside the host cell, the virus is replicated many times by the cells, thanks to the instructions contained in its DNA or RNA, and many copies are made. Basically the cell gets hijacked and turned into a mini-factory to replicate the virus as much as possible.
Let’s look at some real world examples: HIV and Measles.
HIV stands for Human Immunodefiency Virus. It’s transmitted by direct contact of bodily fluids, during unprotected sex or sharing needles. It attacks the patient’s immune system. If too many of the immune system cells are destroyed, the immune system can no longer cope with any infections or cancers.
You might have heard of AIDS. This isn’t a virus, it’s a condition: Acquired Immunodeficiency Syndrome. It’s caused by advanced and uncontrolled HIV infection and, at this stage, the immune system is severely weakened.
However, HIV can be controlled with anti-viral drugs, which stops the virus from replicating. A person can have HIV for many, many years with relatively few symptoms and without developing AIDS due to anti-viral drugs that exist today. And now there are drugs available that people without HIV can take to lower the risk of contracting the virus after possible exposure.
Measles is a very infectious virus, transmitted by droplets spread when an infected person sneezes or coughs. Measles lead to a rash and high temperature and can be serious or fatal if there are complications. Across the world, as many children as possible are given vaccines to protect against measles and to eradicate it from the population.
Next up, bacteria.
So first things first, bacteria are very useful. The bacterial cells inside us are like a hidden organ, helping us to do so many things like breaking down food.
But some bacteria are pathogenic.
These reproduce rapidly and produce toxins that can damage cells and tissue in the body.
Let’s talk about a couple.
Gonorrhea is a sexually transmitted disease caused by bacteria, spread by direct contact when having unprotected sex. Gonorrhea makes urinating painful and causes the production of a thick discharge from genitals. Because Gonorrhea is a bacterium and not a virus, it can be treated with antibiotics and its spread can be reduced by contraceptives like condoms.
Now, salmonella is a bacteria that causes food poisoning, which you can get by eating contaminated food. It can cause stomach pain, vomiting and diarrhoea. To prevent salmonella, poultry are vaccinated against it, and just remember to always cook your food thoroughly. That’s viruses and bacteria done, next we’re going to talk about fungi.
So fungi is a huge category and obviously, not all fungi are pathogenic. We eat mushrooms and use yeast to make bread and beer.
However, some fungi cause disease by penetrating human skin and the surface of plants.
For instance, athlete’s foot is a rash caused by a fungus that lives in people’s toes. You can get it in communal areas like swimming pools, by touching contaminated surfaces. Or you could get it if you share PE socks with someone infected with it. It is treated by anti-fungal medication.
In plants, rose black spot is caused by a fungus that leads to rose plants developing purple or black spots. It can spread through droplets in the air or in water. The leaves turn yellow and can drop off, so this reduces photosynthesis and therefore growth. It is treated by fungicides, which are chemicals that kill fungi. And the fourth and final type of pathogen you need to know are protists.
Protists are single celled, eukaryotic organisms. They aren’t animal cells, plant, fungi, or bacteria, they’re their own thing.
Some protists are parasites that live on or inside organisms and cause them damage.
They often passed on to another organism by a vector, which is just a nice, sciencey way of saying: a carrier of disease that doesn’t get infected. Because the pathogen wants to be able to reproduce, it makes sense that it doesn’t harm the vector.
A good example of this is Malaria, a disease that causes fever, muscle weakness, and can be fatal. It’s spread by mosquitos (so they’re the vectors), and they carry the protist, and spread it when they feed on blood.
Mosquitos suck blood from an infected person which contains the Plasmodium protist. (You won’t need to recall the name of this protist, but it’s good to be familiar with it in case it pops up in your exam!)
Scientists recently have come up with a vaccine for Malaria, but it only really works if it’s given by the age of two. So it helps to stop people from being bitten by mosquitos in the first place. So people sleep under nets and wear insect repellent, and anti-malarial drugs can also help to treat infection.
I’m Dr Alex Lathbridge and this is Bitesize Biology – all episodes available on BBC Sounds
Listen on BBC Sounds
Question
Name a fungal infection of plants and animals.
Athlete's foot is a fungal infection in animals. Rose black spot is a fungal infection of plants.
Episode 2 - Human defence against infection
Dr Alex breaks down the key facts about the different defence systems humans have that help protect against invading pathogens.
Hello, I’m Dr Alex Lathbridge and this is Bitesize Biology.
This is episode two in a six-part series on Infection and Response.
In this episode we’re going to talk about the defence systems that humans have to protect against infection.
In the last episode we looked at the different types of pathogens that can invade our bodies and cells. Thankfully, our bodies have different defence systems to protect us from pathogens.
There are some defence systems that fight against all pathogens
Non-specific defence systems help protect against everything. These non-specific defence systems are either physical barriers or chemical barriers. We're going to look at the skin, the nose, the eyes, trachea, bronchi, and the stomach. So grab a pen and let’s get into it.
First up, one you probably know, the skin.
The skin is your body’s biggest organ. It covers nearly all of you to help protect against invading pathogens.
Imagine it like a huge blanket covering your organs and skeleton and muscles.
If your skin is cut, it can heal itself by forming a scab, so even when there is a hole in the blanket, it can repair itself and acts as a physical barrier from any infections getting in.
Your nose has hairs and mucus which act as a physical barrier to stop invading pathogens. (Yes the hairs and bogies up your nose actually have a purpose!) The hairs and mucus trap pathogens before they can get to the lungs, and so they can be blown out of the nose. The eyes secrete tears, and those tears have enzymes in them, that destroy pathogens and other substances. This is an example of a chemical barrier.
Next is the trachea. That’s the windpipe that runs from your nose towards the lungs. It is lined with cells that have tiny hairs called cilia. The cells waft the tiny cilia hairs, like a Mexican wave, which moves mucus and pathogens upwards towards the throat, where they can be swallowed and moved down to your stomach. This reduces the amount of pathogens entering your lungs.
There are also things known as goblet cells. They’re in your trachea and bronchi and produce mucus which acts as a physical barrier to trap pathogens.
Finally, the stomach. Now this is the big one. It has hydrochloric acid in it, which is strong enough to kill nearly any invading pathogen, in food that’s sent down to the stomach, or ones found in water. This is a type of chemical barrier against infection.
So the physical barriers are the skin, nose, trachea and bronchi.
The chemical barriers are found in the eyes and the stomach.
But what if the pathogens are able to enter your cells? What happens then?
Fortunately, there’s an immune system that has multiple ways to destroy pathogens or limit their effects.
I’m going to take you back over the basics and give you some easy tricks to remember the more difficult bits.
Here’s what you need to know, so grab a pen:
The cells in your body are covered with little molecules known as antigens. These are unique to each of us (unless you’re a twin.) Basically, antigens are ID cards that allow your body to tell your own cells from potentially harmful things that can trigger an immune response.
Cells in your immune system fight anything that they identify as being foreign. That means it's unknown because it has a foreign antigen. Be it nasty things like pathogens or potentially good things like transplanted organs, the immune system mounts a response to things that it sees as foreign.
This is where white blood cells come in.
There are two types of white blood cell that you need to know, phagocytes and lymphocytes.
Phagocytes literally engulf pathogens in a process called phagocytosis, which looks a little bit like a blob eating everything, and then breaking them down using enzymes. They’re like the jack-of-all-trades and can basically deal with anything by engulfing and digesting them.
So if they detect a foreign antigen, that marker, that cell gets broken down, this is known as phagocytosis. Plus, they also signal other phagocytes to come over and help destroy the foreign body.
Phagocytes do this to all foreign bodies, so they are called non-specific. The second white blood cell type that we’re looking at are called lymphocytes.
Unlike phagocytes that engulf and digest non-specifically, lymphocytes are part of specific immune responses (l’ll explain what this means).
There are two main categories of lymphocytes: B cells and T cells.
B cells produce proteins known as antibodies to fight pathogens. These are good, trust me. It sounds weird that they’re called antibodies because it makes it sound like they’re bad for your body but they’re not. I like to think that antibody is just short for “anti-foreign body.”
The key thing to remember is that antibodies are specific to antigens. And remember, each pathogen has its own unique antigen on its surface, that ID card, so each antibody is unique.
These antibodies are complementary in shape to the antigen, like a lock and a key. So that’s how they bind to them.
This allows them destroy pathogens in three ways:
By binding to the antigens on a pathogen, it can stop them from moving by clumping them together.
Then can also call over phagocytes which can destroy them by phagocytosis: engulfing and digesting.
Or the antibodies make the pathogens destroy themselves
But what about your body cells that have already been infected by the pathogen? What happens to them?
Alright but what about your body cells that have already been infected by the pathogen? What happens to them?
Thankfully, some of the lymphocytes stick around in the immune system, as things known as memory lymphocytes.
What this means, is that if you get infected by the same pathogen again, the lymphocytes are able to recognise those antigens and produce high quantities of the correct antibodies very quickly.
So this time the immune response will be a lot quicker and will be able to destroy the pathogen before symptoms arise. This is how vaccination works, but more on that in the next episode.
Two quick things to put this stuff into the real world for you, but you don’t need this for your exams.
First, allergies. If you’re allergic to something, like dust, pollen, or nuts, etc, it means that your immune system is responding to a type of antigen that we call an allergen. Basically, it means your immune system is taking its job way too seriously and fighting off something harmless.
The second thing is that, if someone gets an organ transplant, it can be unsuccessful if the immune system sees the foreign cells as dangerous and starts attacking them. And this is known as organ rejection.
I’m Dr Alex Lathbridge and this is Bitesize Biology. Catch up on BBC sounds.
Listen on BBC Sounds
Question
What molecules do B cell lymphocytes make?
B cell lymphocytes make proteins called antibodies. These are specific to other proteins called antigens found on the surface of pathogens.
Episode 3 - Vaccination, painkillers and antibiotics
Dr Alex breaks down the key facts about vaccination, antibiotics and painkillers.
Hello, I’m Dr Alex Lathbridge and this is Bitesize Biology.
This is episode three in a six-part series about Infection and Response. In this episode we’re going to talk about vaccination, antibiotics and painkillers.
So vaccines are key to having a healthy population. Why are vaccines so important? Well, here’s a little history lesson that’ll make the rest of this episode a breeze, and it’s one of my favourites.
There’s a disease called smallpox, honestly, one of the deadliest diseases known to humans and been around for thousands of years. This isn’t just like chicken pox. We’re talking vomiting, fever, rashes that turned into fluid-filled blisters, scabbing, scarring and even blindness. 1 in 3 people would die, look up smallpox online and you’ll see how bad it is. Well, how bad it was, because smallpox is, as of now, the only human disease to have been completely eradicated.
How? Let’s go back to the 1500s in China. People would pick bits of smallpox scabs from an infected person. They would dry them, grind them up, and blow them into a healthy person’s nostril using a pipe. The idea was that exposing patients to small amounts of the smallpox would make them immune to it later. Similarly in India, they would use a needle to transfer material from smallpox blisters to the skin of healthy children. And West Africans had long practiced the technique and, when enslaved and taken to America, their knowledge was taken with them.
But Europeans were relatively late to the game, only learning about it in the 18th century.
However, instead of using smallpox scabs, a man in the UK called Dr Edward Jenner did something different. He’d heard from communities that milkmaids who got the milder disease of cowpox never got smallpox. So he inserted the pus from a cowpox blister into the arm of a young healthy boy, who felt unwell for about a week or so, but then made a full recovery. Two months later, the boy had matter from a smallpox sore put into his arm and, as Jenner thought, the boy remained perfectly healthy.
This experiment is the one that has gone down in history, meaning that Dr Jenner gets called the father of vaccinations (apparently India and Africa and China don’t count. I hope that your history teachers have taught you that achievements outside of the Western world do get overlooked a fair bit.)
In fact, the word vaccine comes from the Latin word for cow, vacca. Less than 200 years later after his experiment, with advances in medicine, smallpox was completely eradicated.
But why did this work? Let’s break it down, so grab a pen:
Vaccines allow us to be protected from a specific disease in advance, protecting us from becoming ill, if our cells are invaded by that pathogen.
In the last episode, we talked about how our body has defence systems that can protect us from infection. (You should go back and listen if you don’t know) but quick summary: antibodies are proteins produced by white blood cells, known as lymphocytes, to bind to specific antigens found on the surface of foreign cells (these are like ID cards to show which cells are yours and which are not).
After infections, memory lymphocytes are stored in the body (which is like having a database of pathogen ID cards) and so if you get infected by the same pathogen again, you can very quickly mass-produce the correct antibody. This makes us immune so the pathogen can’t infect us.
So vaccines allow us to do the same thing, in a more controlled way. Basically, it’s like having a tutorial level of a game, or instead of playing online with your friends, playing against bots.
Instead of blowing scabs up your nose or pricking you with pus, a small amount of a dead or inactive pathogen enters your body, usually via injection.
The pathogen still has its antigens all over so, despite it being weak or dead, the immune system still gets triggered, and it is GO TIME.
So first up, your lymphocytes produce the specific antibodies which target and bind to the antigen. Other white blood cells called phagocytes then engulf and digest the pathogens (remember: this is phagocytosis).
And then memory lymphocytes that make the antibodies are stored in the spleen for many years. So if you get exposed to the live version of the same pathogen, your lymphocytes can produce lots of antibodies really quickly and destroy the pathogen easily. Meaning you aren’t going to get ill and are immune.
Remember, pathogens have their own specific antigens (those ID cards). Antibodies are very specific to one pathogen’s antigens. So a vaccine for the virus that causes Measles can’t also protect against the flu virus.
Speaking of which, some vaccinations, like the one that you have for the flu virus, wear off over time. I get my flu jab every year to stay immune. Basically, it’s because some viruses replicate really fast and can mutate into new strains (remember not species, because viruses aren’t alive like bacteria.)
And with a new strain comes new antigens, so new antibodies are required. Whereas other viruses, like smallpox or measles, are more stable and less likely to change.
But health doesn’t start and end at one person, it’s about community.
The majority of a population needs to be vaccinated against very serious diseases, like smallpox, as this means people are less likely to come into contact with disease-causing pathogens. This helps protect people who are more at risk or who are unable to be vaccinated.
This concept of a population being protected against a specific disease by a high percentage of them being vaccinated is called herd immunity.
Alright, that’s vaccines over but there aren’t vaccines for every type of pathogen. However, there are different types of medicines that can treat diseases: painkillers and antibiotics.
Painkillers are chemicals that reduce symptoms from pathogens but they don’t kill the pathogens themselves. It’s in the name: pain killer. These drugs help you deal with the symptoms of the illness, such as headaches, that occur while your immune system is fighting the pathogens.
Now, antibiotics are drugs that kill or stop the growth of one type of pathogen: bacteria. They don’t work on viruses, like flu, only bacterial diseases.
Penicillin is a great example of an antibiotic. They destroy bacterial cells without killing your own body cells.
Antibiotics target specific bacteria, so it's important that you get prescribed the right antibiotic.
Since the introduction of antibiotics they had have a huge influence on the health of the world’s population. Lots of bacterial diseases that previously killed people are now able to be treated with antibiotics.
But there is one problem though: antibiotic resistance.
No, that isn’t the bacteria rioting or something. It’s when bacteria randomly mutate and become resistant to some antibiotics.
This happens in three ways:
Overuse of antibiotics, especially for conditions that don’t need them.
Patients not taking the full course of antibiotics that they’ve been prescribed, so some bacteria aren’t destroyed and have the opportunity to mutate
Antibiotics are overused in farming, to prevent disease in animals, but this can lead to resistant bacteria building up in the environment and spreading.
All of this behaviour combined can increase the likelihood of mutations leading to resistance, meaning that infections and diseases that we can treat today, might not be treatable in the future.Because recently we’ve not found many new antibiotics.
MRSA is a type of bacteria resistant to more than one type of antibiotic, so it's really hard to treat.
Viruses are not cured by antibiotics, so scientists develop anti-viral drugs to treat viruses.
But it is really difficult to develop successful anti-viral drugs, as viruses hide inside cells so they are hard to treat.
Before you go, I have a personal plea:
What I really want you to take away from today is that it’s down to all of us to change the way we think about antibiotics. Because if we can limit their use to only when they’re necessary, and everyone makes sure that they do finish a course of antibiotics when they’re given them, we can keep the diseases of today treatable and make it easier to find even better treatments for diseases in the future.
I’m Dr Alex Lathbridge and this is Bitesize Biology – all episodes available on BBC Sounds
Listen on BBC Sounds
Question
What type of diseases do antibiotics treat?
Antibiotics are only used to treat bacterial diseases. They cannot be used for diseases which are caused by viruses, protists nor fungi.
Episode 4 - Drug discovery
Dr Alex examines the key facts about drug discovery and the stages of testing that potential new drugs go through.
I’m Dr Alex Lathbridge, and this is Bitesize Biology.
This is the fourth episode in a six-part series about Infection and Response. In this episode we’re going to talk about drug discovery: how new drugs are discovered and how they are tested before they can be used by you and me.
And just a heads up, in this episode we’re going to be talking about testing on animals.
Lots of our current medicines were discovered by studying plants. And that sounds weird, right?
Well, not when you think about history and biology. Because plants are organisms that can get diseases, just like us. So they’ve evolved to have chemicals that protect them against infection.
And before modern medicine made pills and injections commonplace, people would find ways to treat themselves, so they looked to different plants.
For thousands of years, plants have been used to treat illnesses and their symptoms. Because they contain hundreds of different chemicals, with some of them being useful for drugs.
Today, there are over a hundred active compounds derived from plants for use as drugs and medicines. For instance, quinine found in tree bark is the basis for the first anti-malarial drugs. And chemicals taken from pacific yew trees have been used as the basis for chemotherapy drugs.
It’s not that you can just eat the plant or brew it into a tea, as they did in the past. Nowadays, scientists have been able to extract the active compounds, the chemicals that protect them against infection, and work out how they can be turned into medicine.
You’re going to need to remember these examples for your exam:
The Ancient Greeks used willow bark to help cure fevers and pain. It was later discovered that it had an active ingredient, salicylic acid, and this same ingredient is now used in the modern painkiller Aspirin.
The drug Digitalis is used to treat heart conditions. This was originally developed from the flowering plants known as Foxglove, (which is the Latin name for the genus Digitalis). But they also have other names such as Dead Man’s Bells and Witches Glove. Why? Because, depending on the species of Foxglove plant, the chemicals inside can be toxic. This is a good example of why it's important to understand how the chemicals inside plants work, because it allowed scientists to work out the correct compound and dosage required to be used as medicine.
Some drugs have been extracted from microorganisms, a famous one is penicillin. Penicillin was the first antibiotic discovered back in 1928 by Alexander Fleming.
He discovered it almost by chance. He was a bit of a sloppy scientist and went away on holiday leaving a sandwich out in his lab. When he came back, he noticed some petri dishes that had developed bacteria and mould from the sandwich. The area around the mould was completely free of bacteria. He found that the mould was a naturally occurring substance, that killed the bacteria, this became known as penicillin.
Today drugs are created by scientists in laboratories, but they may still start with a chemical extracted from a plant.
So let’s look at drug discovery.
It’s really important that when new potential drugs are found that they are tested thoroughly to make sure that they’re safe. An example of when this didn’t happen is the story of a drug called Thalidomide
Thalidomide is a drug that was originally developed in the 1950s as a sleeping pill but was also thought to be useful for pregnant women to reduce morning sickness. Unfortunately, it had not been tested thoroughly for use in this way and caused unexpected side effects and serious damage to unborn babies.
By 1960 Thalidomide was found to damage the development of unborn babies, leading to arms or legs of the affected babies being very short or incompletely formed. More than ten thousand babies were affected around the world and it was banned for use. It caused major changes in pharmaceutical policies worldwide, including a rule that, if a drug was to be taken when pregnant, they had to be tested to see if they were safe for use in pregnancy.
Let's take a look at how testing a new drug works.
Imagine that you and I are in the lab, and we’ve got to test new potential compounds before they can become drugs to be prescribed.
Grab a pen and write this down:
There are three things that we need to test potential compounds for:
Their safety. Some drugs might be toxic of have harmful side effects
Their effectiveness, also known as efficacy. Basically, how well a drug cures a disease or helps with symptoms
The dosage, what the optimum dose for humans is. This one is basically a balance of the last two. You want to make sure that the dosage is high enough to be effective but not so high that it causes dangerous side effects.
There’s a really good saying from the 1500s that sums this up and is worth remembering: the dose makes the poison.
So the important factors are: safety, effectiveness, and dosage.
So we’re in the lab right now, how do we get that data?
This can also be broken down into three stages. There are three stages of testing new potential drug compounds :
Testing on Cells and Tissues. Compounds are initially tested using human cells and tissues grown in a lab. This allows for efficacy and possible side effects to be tested. You can tell which new compounds fail this first step because they damage cells (which are side effects) or do not work (which is efficacy, or effectiveness). Once we have a compound that’s successful from there, we move on to the next step.
Testing on animals. This involves giving a known amount of the compound to an animal, and then checking for any side effects. We can check the efficacy and how safe it is this way.
The law in the UK is very strict that any new drug must be tested on two different species of live animal. There are real ethical considerations with testing new drugs on animals.Not all scientists have the same opinion and this is something that opens up lots of debate
So once animal testing is completed, successful compounds move onto the third and final stage.
- Testing on human volunteers. Compounds are tested in two ways: first in healthy volunteers to check they are safe and give no worrying side effects. Then the same drug is then tested on those who already have the illness, to check that it is safe and actually works. At the start, doses of the drug are very low, and then gradually they get increased to find the optimum dose. I don’t know about you, but when I was in primary school and someone scraped their knee playing, the school nurse would treat it with some blue tissue and water. We were told though, that it was magic tissue and magic water and we immediately felt better.
This was my first discovery of something known as the placebo effect: essentially the belief that something will have a positive effect on you.
A placebo is a tablet or medicine or treatment that has no active drug in it. These are used in drug testing with humans, where patients are split into two groups. One group is given the placebo (which has no drug in it) and the other group is given the new drug being tested.
Double-blind trials are often used to test drugs where neither the patient neither the doctor doing the tests, knows whether the patient in the study is getting the drug or the placebo. This is so the patients and the doctors aren’t subconsciously influenced, and the drug test is as unbiased as possible.
I’m Dr Alex Lathbridge and this is Bitesize Biology – subscribe on BBC Sounds
Listen on BBC Sounds
Question
Historically, where did most of our drugs come from?
Drugs have historically come from nature, as parts of plants or microorganisms. The heart drug digitalis comes from foxgloves. Penicillin, the first antibiotic, was discovered by Sir Alexander Fleming in mould.
Episode 5 - Monoclonal antibodies
Dr Alex explores the key facts about how monoclonal antibodies are made in a laboratory.
Hello. I’m Dr Alex Lathbridge and this is Bitesize Biology.
This is the fifth episode in a six-part series on Infection and Response. In this episode we’re going to talk about Monoclonal Antibodies.
A quick heads up: today we are going to be talking about testing on animals.
This technology helped win the Nobel Prize in 2018 because scientists found a way to use it to treat cancer.
It’s the reason why scientists in the lab don’t have to cover fish in urine (this is relevant later) and I am willing to bet good money that you’ve seen monoclonal antibodies in the shops without even realizing it.
By now, we’re good on the basics of antibodies? (If not, no worries, go back to episode 2 of this series if you’re really stuck) but here’s a quick recap:
Antibodies are very specific. They work by binding onto the specific antigens found on the surface of pathogens. So only one type of antibody will bind to one type of antigen.
But here’s where it gets cool.
Remember how we’ve been describing antigens so far? They’re like ID cards for every cell, not just pathogens.
Well, scientists worked out that it was possible to make antibodies that could identify and bind to antigens on almost anything, not just pathogens.
This is where Monoclonal Antibodies come in and chances are you’ve seen them in use without realising it, so grab a pen and write this down:
First up: Monoclonal. Mono means one. Clonal means clone.
In easy terms, monoclonal antibodies are created by exposing the immune system to a specific antigen and when the antibodies get generated, scientists use cloning techniques in the lab to make lots and lots of them, basically letting us store lots of one specific antibody. Hence the term, monoclonal antibodies.
Let’s say we’re in the lab and we want to make a batch of monoclonal antibodies to target an antigen found in something like peanuts, for people with peanut allergies. Peanuts have lots of different antigens so we’ll just pick one for now.
So this is going to involve live mice, spleen cells, and a cancer cell. Obviously don’t do this at home.
Let’s get into the five steps you need to know to make a monoclonal antibodies:
- First thing, you get your mouse and vaccinate it by injection with a specific antigen (in our
case, this is an antigen found in peanuts)
The mouse naturally responds to the peanut antigen, makes lymphocytes which produce antibodies that specifically target our injected peanut antigen. So we’ve got ourselves some antibody producing lymphocytes, but it’s slow.
We do a little operation on the mouse to remove spleen cells which contain these lymphocytes.
These spleen cells are then combined with human cancerous cells, which are called myeloma cells, this combination are called hybridoma cells. Literally hybrid myeloma. Hybridoma cells.
These hybridoma cells divide indefinitely and reproduce rapidly, making clones of themselves and creating millions of monoclonal antibodies specific to the original antigen. These hybridoma cells make the peanut antibody that we want and that’s what we need.
I know your first question: why on earth have we just combined spleen cells and cancerous cells? That doesn’t sound good.
Fair question and that is what is really clever about this.
So remember what are cancers caused by? Cells that divide rapidly and uncontrollably.
So what we’ve done is say “let’s turn that negative into a positive.”
So instead of waiting for lymphocytes to divide in the spleen, let’s combine those spleen cells with cancerous cells that we know divide really quickly, and that combination – the hybridoma – will churn these monoclonal antibodies out like a factory.
So that means that we can pick and choose which specific antibodies to make and make a whole lot of them.
That monoclonal antibody that we just made to target an antigen found in peanuts could theoretically be used to help people who get terrible allergic responses to them.
So let’s take monoclonal antibodies out of the lab and into the real world.
Now remember, I said you’ve probably seen monoclonal antibodies in shops without realising it?
That is because they are the basis of pregnancy tests. Yes, the stick that people urinate onto that changes colour depending if they’re pregnant or not.
Why? Well, a hormone called HCG is present in the urine of pregnant women. Monoclonal antibodies are used in pregnancy tests by binding specifically to HCG, causing the colour change, so someone can find out whether they are pregnant or not.
It’s a good thing we have monoclonal antibodies now because before home testing kits were invented in the 1970s, scientists used animals, such as certain toads and fish to test for pregnancy. Women’s urine would be put in the water of a specific type of see-through fish. If HCG was present in the urine, one of its organs would expand and this would confirm pregnancy. And you could see it because it was a see-through fish.
How can monoclonal antibodies be used to diagnose and treat cancer?
Antigens our cells’ ID cards, so it makes sense that cancerous cells have their own specific antigens.
Monoclonal antibodies can be designed to specifically bind to these antigens on the cancerous cells, and clump them together.
This is good because they have the potential to:
identify a cancerous tumour, so it can help with possible treatment or removing the tumour.
they can help carry drugs to target the specific cancerous cells.
they can encourage your immune systems to attack the cancer cells.
We’ve been very positive about monoclonal antibodies and like I said, this technology helped win a Nobel Prize.
But there are some pros and cons. Let’s start with the pros:
Monoclonal antibodies can be produced very quickly even though it can be initially time-consuming when they are made for the first time
They only bind to specific antigens or target cells and they do not affect any other healthy cells, and that’s good.
But there are cons:
Monoclonal antibodies are very expensive to produce.
There are ethical concerns about using animals in the lab.
Scientists originally thought that monoclonal antibodies would be a “magic bullet” and identify and treat many medical conditions. But tests have shown that unfortunately this is not the case. The human body is super complicated and some interactions with monoclonal antibodies get unwanted side effects, and so they cannot be as widely used as doctors first thought.
I’m Dr Alex Lathbridge and this is Bitesize Biology. You can listen to all episodes now on BBC Sounds.
Listen on BBC Sounds
Question
What two cells are joined to make a hybridoma cell?
A hybridoma cell is formed when a spleen cell which produces lymphocytes is fused with a myeloma (cancer) cell.
Episode 6 - Plant disease
Dr Alex breaks down the key facts about plant disease and the physical and chemical defences that protect plants against pathogens.
Hello, I’m Dr Alex Lathbridge and this is Bitesize Biology.
This is the final episode in a six-part series about Infection and Response. In this episode we’re going to talk about plant disease and what physical and chemical defences plants have against pathogens. We’ve already spoken about the four different types of pathogens that cause disease: viruses, bacteria, fungi and protists. If that doesn’t sound familiar, go back to episode 1 of this series on BBC Sounds and take a listen.
Remember: pathogens have very simple life cycles: they infect a host, reproduce many times (or replicate if they’re a virus) and then leave their host to infect other cells or organisms.
So far we’ve mostly talked about what happens when pathogens invade the cells and bodies of humans. When pathogens invade the cells of plants, they can reduce their growth or even kill them.
And that can lead to a reduction in biodiversity. To be clear, that’s bad.
Pathogens that target plants that humans use for food crops are especially serious, for example the Irish Potato Famine in 1850, was caused by a mould that destroyed tomatoes and potatoes, and this lack of food led to starvation that killed over one million people. This is important stuff.
Let’s take a look at two examples of pathogens that target plants, you need to know the names of these, and how they are spread and what the treatments are. So grab a pen:
First up, the Tobacco Mosiac Virus. This was the very first virus to ever be identified. This affects the tobacco plant (as well as other closely related species like tomatoes and peppers.) It’s transmitted by direct contact from one plant to another, either naturally or spread on the hands of farmers. It infects the chloroplasts of plant leaves and leaves a mosaic pattern on the leaves, hence the name. So what it does is it discolours their leaves, and that reduces the amount the plant can photosynthesize. This has a knock-on effect on reducing the plant’s growth, and so farmer’s yields, the amount that they can produce, are reduced too.
A second example is rose black spot, which is caused by a fungus. It can spread through the air, in water or through direct contact on gardeners’ hands. Rose plants develop purple or black spots. The leaves can turn yellow and then they can drop off, reducing photosynthesis and growth. It can be treated through fungicides, which are chemicals which kill fungi, and by destroying any infected leaves.
But pathogens aren’t the only things that damage plants. Let’s not forget about insects and the damage they can do.
You might have heard of something known as aphids. Aphids are tiny greenflies that infect rose and tomato plants. They are found in large numbers on the shoots and stems of plants and they suck sap out of them. Plants need water and nutrients to survive so sucking this sap out slows the growth of plants and can eventually kill them.
To get rid of aphids, gardeners often try to have lots of ladybirds in their gardens, because ladybirds like to eat aphids, and this is a natural way of getting rid of them, rather than using chemicals.
Now let’s take a look at the defence systems of plants. Because, like humans, plants have both physical and chemical barriers. Remember these three physical defences of plants:
Trees have bark, which is actually a layer of dead cells which surrounds the plants to form a physical barrier against infection.
Each plant cell has a cellulose cell wall which is another tough barrier that protects against pathogens.
Leaves are covered in a waxy cuticle, which is like a protective layer of waxy skin. The leaves are where the all-important photosynthesis happens so it’s important to keep the cells in this area protected with a physical barrier. And there’s also these chemical defences:
Humans have our enzyme-filled tears and stomach acid, plants have something a bit different.
Plants like mint and witch hazel produce antibacterial chemicals (a bit like antibacterial handwash that we use.) These reduce the spread of bacteria that get past the physical barrier. Stinging nettles have developed poisons to stop them being eaten by herbivores (animals that only eat plants). If you've ever been stung by stinging nettles, you can imagine why that sting would put off an animal eating them. But the poison from stinging nettles can only get them so far, the poison doesn’t help with infection from pathogens.
And also remember chili peppers. The reason why they make your mouth burn is because of a chemical called capsaicin. This affects mammals but birds don’t feel that burn. Their mouth doesn’t have the receptor to feel the chemical. This evolved to prevent mammals from eating them but birds have no problem, because birds fly can really really far and poop out the plant seeds, thereby spreading them far and wide, which is a good thing.
Another thing to remember is that farmers can now genetically engineer plants that are resistant to disease. We’ll be talking about that process in much more detail in our series on inheritance, variation and evolution. So that’s physical defenses and chemical defenses. But unlike humans, plants also have mechanical defences.
What does that mean? Have you ever tried to eat a rose? Roses are covered in thorns and hairs. Likewise, cacti have thin spines. These adaptations stop the plants from being eaten and damaged, so that’s one mechanical defence.
A second mechanical defense is drooping leaves. The mimosa pudica plant has evolved the ability to close its leaves and point its stems down towards the ground, like limp spaghetti whenever an insect lands on it, which then makes it really difficult for the insect to then eat the leaves.
Finally, mimicry. Some plants have evolved the ability to mimic (or look like) their surroundings, to stop themselves from being eaten. The passion flower vine has small yellow spots on its leaves, which look really similar to butterfly eggs. Butterflies can’t tell the difference between real eggs and the passion flower leaves, so they lay eggs on leaves away , to avoid competition with these pretend eggs. Because no eggs are laid on them, the passion flower leaves are less damaged because no feeding caterpillars are born on them. I’m Dr Alex Lathbridge and this is Bitesize Biology. All episodes available now on BBC Sounds.
Listen on BBC Sounds
Question
Why do some gardeners encourage ladybirds into their gardens?
Ladybirds are natural predators of aphids which can infest plants like roses and tomatoes. Aphids suck sap from plants which weakens them.
Links to further learning for science GCSE
More on Podcasts
Find out more by working through a topic
- count4 of 7
- count5 of 7
- count6 of 7
- count7 of 7